Extracellular vesicles (EVs) play a significant role in intercellular communication and are known to function in maintaining homeostasis and driving disease progression in the body. Exosomes, a specific type of EV, are small vesicles with an average size of about 100 nm and possess a bilayer membrane structure similar to the plasma membrane. Exosomes originate from endosomes and contain various internal molecules. Because they are generated within cells, the substances they carry often reflect the characteristics and state of their originating cells. Owing to these properties, exosomes can provide insights into the state of cells or tissues, making them a promising biomarker for disease diagnosis. Additionally, by using therapeutic donor cells or modifying their internal cargo, exosomes can be engineered for therapeutic purposes.
This article is the first in a series aimed at providing a comprehensive understanding of EVs and exosomes. While numerous papers on EVs have been published, this series will reference a paper from Science published when the concept of EVs was beginning to take shape, to clarify the foundational concepts.
Extracellular Vesicles and Their Classification
Despite the diagnostic and therapeutic potential of EVs in conditions such as neurodegenerative diseases, cardiovascular disorders, and cancer, their biogenesis, purification methods, and classification remain incompletely understood. Current EV activity evaluations are limited to vesicles obtained from cell cultures rather than those produced under physiological conditions. Additionally, due to incomplete purification methods and low EV purity, these evaluations cannot be considered fully accurate. Furthermore, experimental limitations, such as the difficulty of single-particle observation or tracking, pose challenges in understanding the physiological roles and characteristics of EVs.
Nonetheless, growing evidence suggests that EVs are not merely cellular waste disposal tools but are deeply involved in essential physiological processes, such as signaling the state of cells and tissues and maintaining homeostasis. Although the concept of EVs is still being refined, the referenced paper broadly classifies EVs into two categories: exosomes and ectosomes.
Ectosomes are vesicles secreted through budding from the plasma membrane, with microvesicles being one example. While microvesicles can share size similarities with exosomes, their particles can be as large as 1 µm. In contrast, exosomes originate from endosomes and predominantly range in size from 40 to 160 nm.
However, as previously mentioned, there are no established purification or analytical methods to isolate exosomes exclusively. This means current research often involves studying EV populations rather than pure exosomes. As such, the traits attributed to exosomes might actually describe features of mixed EV populations. It is crucial to acknowledge that the observed characteristics of exosomes and the proteins defined as their markers may represent only specific subpopulations of exosomes or mixed vesicles with diverse phenotypes.
This article will focus on exosomes and explore their specific properties in comparison to other vesicles.
The Biogenesis of Exosomes
Exosomes are formed through a two-step invagination process from the plasma membrane, leading to the formation of multivesicular bodies (MVBs), which contain numerous intraluminal vesicles (ILVs). These ILVs, which are later secreted as exosomes, are vesicles typically 40-160 nm in size and are released when the MVB membrane fuses with the plasma membrane.
The first step involves invagination of the plasma membrane, forming a cup-shaped structure that encapsulates cell surface proteins and soluble proteins from the extracellular environment. This results in the formation of early-sorting endosomes (ESEs), which may merge with pre-existing ESEs. During this process, the interaction between the Golgi apparatus and the endoplasmic reticulum determines the internal cargo of the ESEs. The ESEs then mature into late-sorting endosomes (LSEs) and eventually form MVBs. The second invagination occurs during MVB formation, where the endosomal membrane invaginates inward to create ILVs. This marks the final invagination process, resulting in MVBs containing multiple ILVs that will later be secreted as exosomes.
MVBs can either fuse with lysosomes or autophagosomes to degrade their contents or merge with the plasma membrane to release ILVs as exosomes. Proteins such as Rab, Syntenin-1, TSG101, ALIX, Syndecan-1, and ESCRT proteins, as well as lipids such as phospholipids and ceramides, play significant roles in the biogenesis and secretion of exosomes.
However, the exact mechanisms of exosome biogenesis remain unclear due to the overlap with other vesicular transport pathways. For example, experiments inhibiting ESCRT proteins have shown indirect effects, such as altering lysosome or autophagosome function and disrupting Golgi-derived material transport, all of which could influence exosome biogenesis. Additionally, factors such as cell type, culture conditions, and metabolic state are thought to play critical roles in exosome production. Differences in exosome isolation, concentration, and purification methods across experiments may also contribute to the variability in results. Consequently, the precise mechanisms governing exosome formation have yet to be fully elucidated.
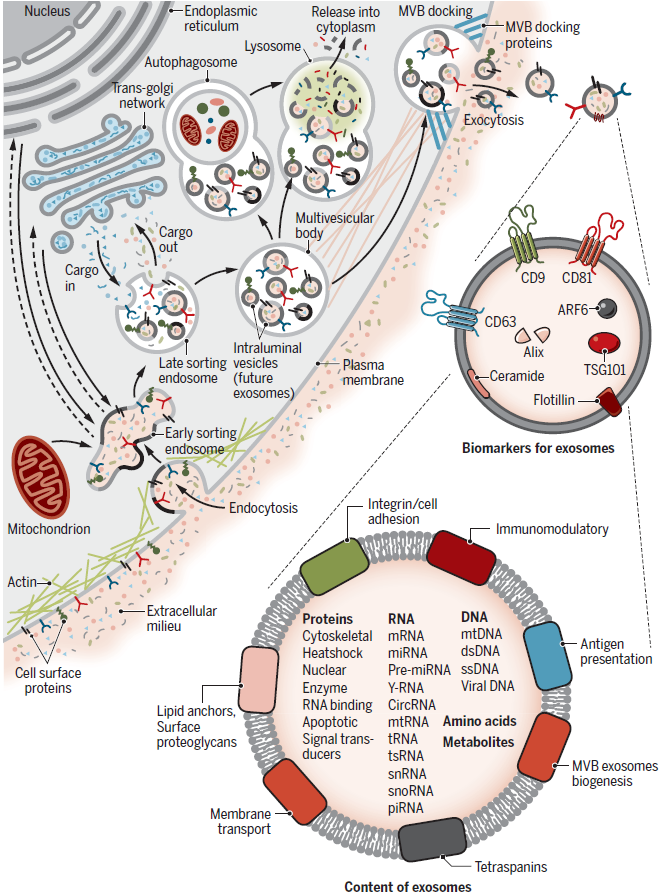
Heterogeneity of Exosomes
The heterogeneity of exosomes arises from differences in their size distribution, cargo content, functional effects on target cells, and cellular origin. Variations in size may result from uneven invagination during MVB formation, leading to differences in total cargo content, or from isolation methods that include other types of EVs. The cargo of exosomes consists of membrane proteins, cytoplasmic or nuclear proteins, extracellular matrix proteins, metabolites, and nucleic acids (mRNA, noncoding RNA, DNA). These materials are not evenly distributed across all exosomes, contributing to size variability.
For instance, proteomic analysis of EVs has revealed that common exosome markers do not exist in identical amounts or ratios in all exosomes, highlighting the need for caution when designing purification experiments using conventional markers. Nevertheless, studies using proteomics and nucleic acid profiling have demonstrated that exosomal cargo reflects the characteristics of their originating cells. This suggests that exosome formation is not a random process, but rather involves specific cargo-loading mechanisms for proteins and nucleic acids.
Additionally, functional heterogeneity arises because the properties of exosomes depend on the type of their originating cells. For example, data suggest that the organotropism and uptake patterns of exosomes administered in vivo are determined by their cell of origin. The diversity of factors influencing exosomal heterogeneity—such as cell origin, size, and cargo composition—creates a complex and multidimensional landscape of variability.
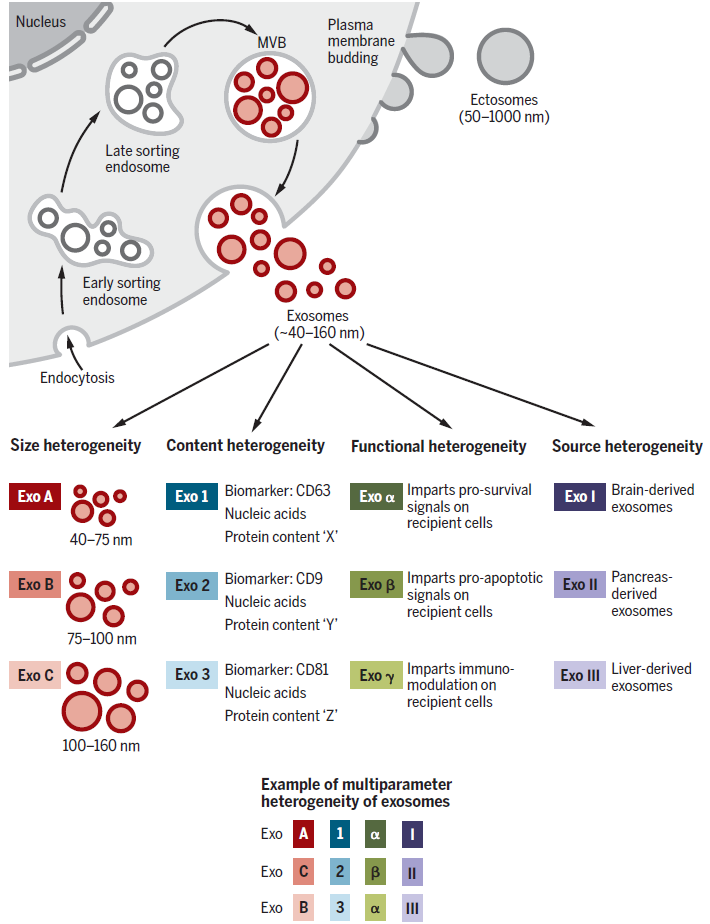
Intercellular Interactions
The mechanisms by which exosomes are taken up and their unique characteristics depending on their cellular origin add complexity to their roles in intercellular communication. For example, oncogenic signals induced by mutant KRAS in pancreatic cancer cells promote the uptake of exosomes via macropinocytosis—a process in which the plasma membrane forms protrusions that engulf exosomes, followed by vesicle formation. In contrast, melanoma cells absorb exosomal cargo through membrane fusion, while PC12 cells of adrenal medulla origin rely on clathrin-dependent pathways for exosome uptake. However, it remains unclear how these different uptake mechanisms influence the distribution, digestion, and functional expression of exosomes post-uptake. Moreover, differences in organotropism based on the origin of exosomes also remain under investigation.
Proteomic studies of exosomes suggest that their protein composition can induce physiological changes in target cells. For instance, tumor progression involves changes such as the activation of EGFRv3 in glioblastoma cells, which secrete exosomes rich in pro-invasive molecules. Neural stem cells exposed to inflammatory cytokines produce exosomes containing IFN-γ-bound IFNGR1, which, upon uptake by recipient cells expressing IFNGR1, activates the STAT1 signaling pathway. These examples demonstrate that exosomal proteins selectively activate specific signaling pathways, influencing processes such as development, immune responses, and disease progression.
This article has provided an overview of EVs and focused on the biogenesis and characteristics of exosomes, one of their subgroups. While exosomes are gaining attention as therapeutic agents and diagnostic biomarkers, their formation mechanisms and cargo-loading processes remain insufficiently understood, underscoring the need for further research. The reference paper for this discussion was published in 2020, and while more findings have since emerged, significant gaps in knowledge persist. Nonetheless, elucidating the mechanisms underlying exosome formation and cargo loading could vastly expand their potential applications.
In the next installment, we will delve deeper into the roles of exosomes in vivo, their functions in disease progression, and strategies for harnessing them in therapeutic approaches.
Reference : Kalluri R, LeBleu VS. The biology, function, and biomedical applications of exosomes. Science. 2020 Feb 7;367(6478):eaau6977.