As research continues to reveal the impact of the tumor microenvironment (TME) on cancer treatment, approaches that focus on altering the TME, rather than directly targeting the tumor itself, are emerging as effective strategies for cancer therapy. In this article, we will explore the relationship between macrophages, a key component of the TME, and cancer treatment.
The TME provides the essential conditions for tumor development and growth, with inflammatory cells and their mediators serving as its core components. Tumor-associated macrophages (TAMs) act as a crucial link between inflammation and cancer. The formation of an inflammatory tumor microenvironment can generally be attributed to genetic factors or inflammatory triggers from the external tissue environment. However, these causes can vary or differ depending on the type of tumor tissue. Despite this variability, the infiltration of myeloid monocytes, such as monocytes or macrophages, into tumors is a common characteristic across all types of tumors, regardless of origin or location.
TAMs are characterized by their plasticity in response to environmental conditions, making them a double-edged sword from a therapeutic perspective. When functioning in a tumor-suppressive manner, TAMs can induce tumor cell death, mediate antibody-dependent cellular cytotoxicity or phagocytosis, inhibit angiogenesis, promote tumor necrosis, and activate leukocytes to elicit an anti-tumor response. Conversely, in more established tumors, TAMs can drive tumor progression and metastasis through various mechanisms.
In today’s article, we will focus on the roles and types of TAMs, examining how they influence conventional cancer treatment methods.
◈ The Diversity of TAMs
TAMs primarily differentiate from bone marrow-derived monocytic precursors. However, under homeostatic conditions, tissue-resident macrophages (TRMs), which originate from embryonic precursors, also appear to play a role in maintaining TAM levels.
A common feature of TAMs across various types of cancer is their role in influencing tumorigenesis and development through immunosuppressive mechanisms. In pancreatic cancer, TRMs have been observed to increase locally and contribute to tissue fibrosis, as suggested by their transcriptional profiles. In a mouse model of ovarian cancer, peritoneal macrophages were found to support the preservation of cancer stem cells, thereby promoting metastasis. In the brain, which is separated by the blood-brain barrier (BBB), brain-specific macrophages known as microglia were shown to promote the proliferation and metastasis of brain tumor cells through immunosuppressive mechanisms mediated by mTOR signaling. Epigenetic changes occurring during these processes have been reported to activate the recruitment of TAMs to the tumor microenvironment, thereby transforming surrounding tissues into a tumor-friendly environment.
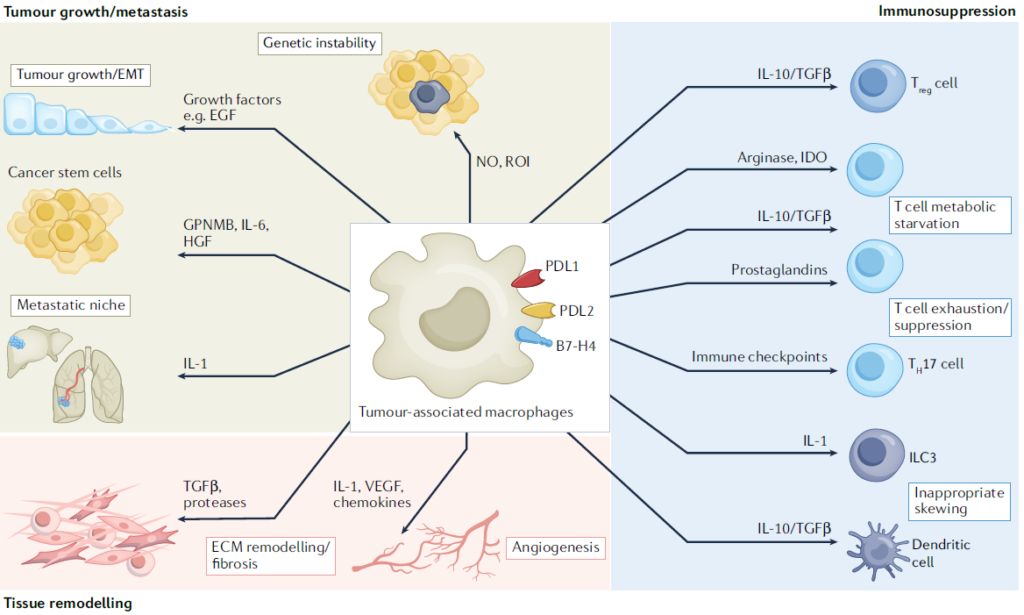
From a genetic perspective, TAMs are predominantly composed of TRMs, which appear to play a role in protecting tumor cells from the immune system during the early stages of tumor development. As the tumor progresses and grows, bone marrow-derived monocyte macrophages are recruited to the tumor site, accelerating tumor growth. This has been observed in models such as murine glioma. However, in contrast, lung cancer models have shown that both TRMs and bone marrow-derived monocyte macrophages maintain the number of regulatory T cells (Tregs), contributing to the formation of an immunosuppressive TME in the early stages of the tumor. Thus, macrophages act dynamically within the TME and play different roles depending on tissue specificity, although in most cases, they are known to positively contribute to tumor growth.
While TAMs can be broadly classified into two groups based on their origin, using techniques like single-cell RNA sequencing allows for the collection of more detailed subpopulation information, which can help in refining therapeutic targets. TAMs typically exhibit an M2-like phenotype. They are recruited to the tumor site or differentiated into an M2-like phenotype through signals such as TGF-b, CSF1, CCL2, IL-4, and IL-1 secreted by various immune cells, as well as histamine or substances derived from gut microbiota that bias them towards tumor-promoting activity. However, as mentioned earlier, single-cell analysis reveals that TAMs possess a variety of sub-phenotypes, transcriptomes, and cell surface proteins, leading to different sensitivities to drugs or therapies. Therefore, by using single-cell analysis to gather data on the various TAMs present in different tissue-specific cancers, innovative therapeutic approaches can be developed, and preventive diagnostic markers can be discovered.
Given the plasticity of TAMs and the lack of precise understanding of the causes that lead to functional changes in TAMs, it is challenging to hastily target TAMs for therapy. However, as research progresses on the negative prognostic implications of macrophage infiltration in tumors, clinical evaluations of macrophage-targeting therapeutic strategies are gradually advancing.
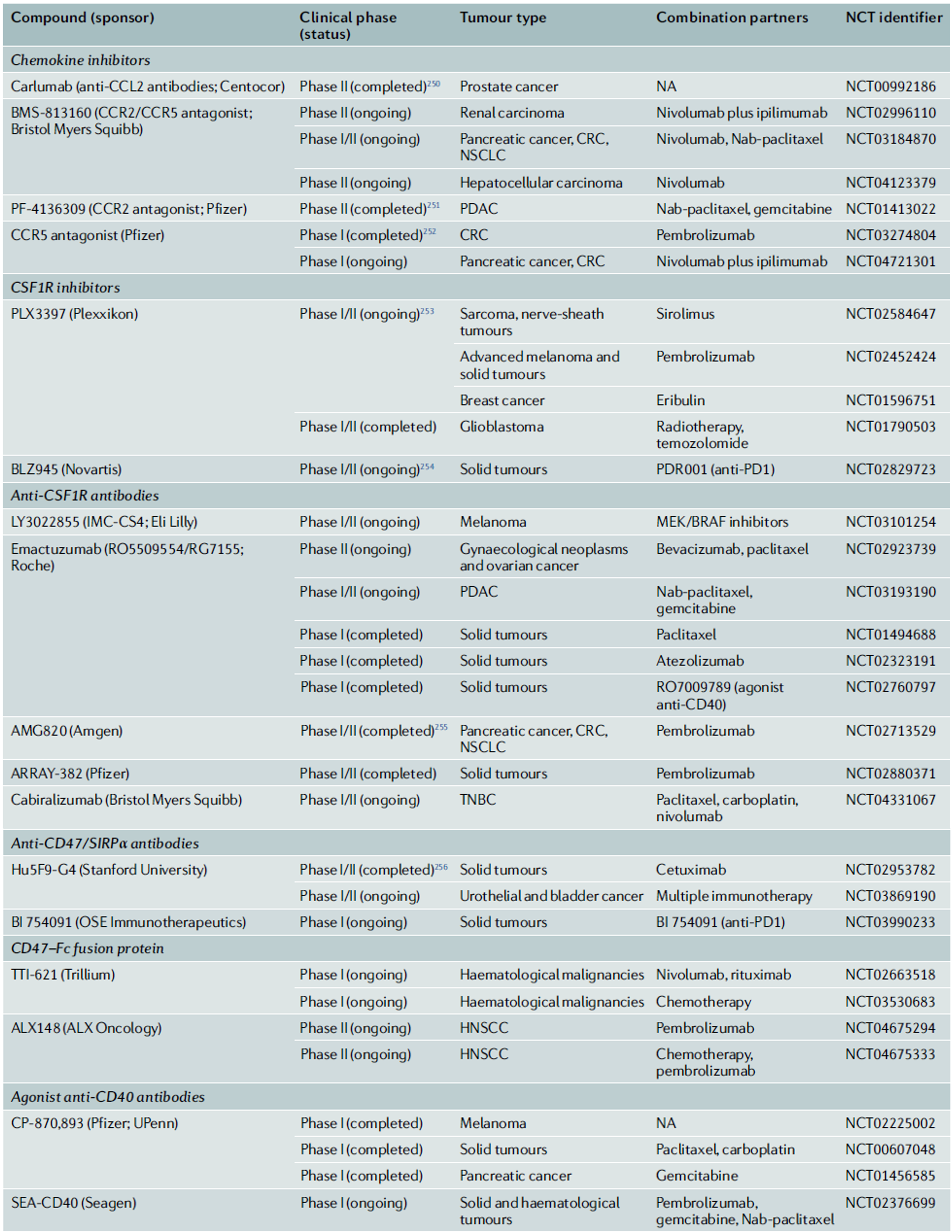
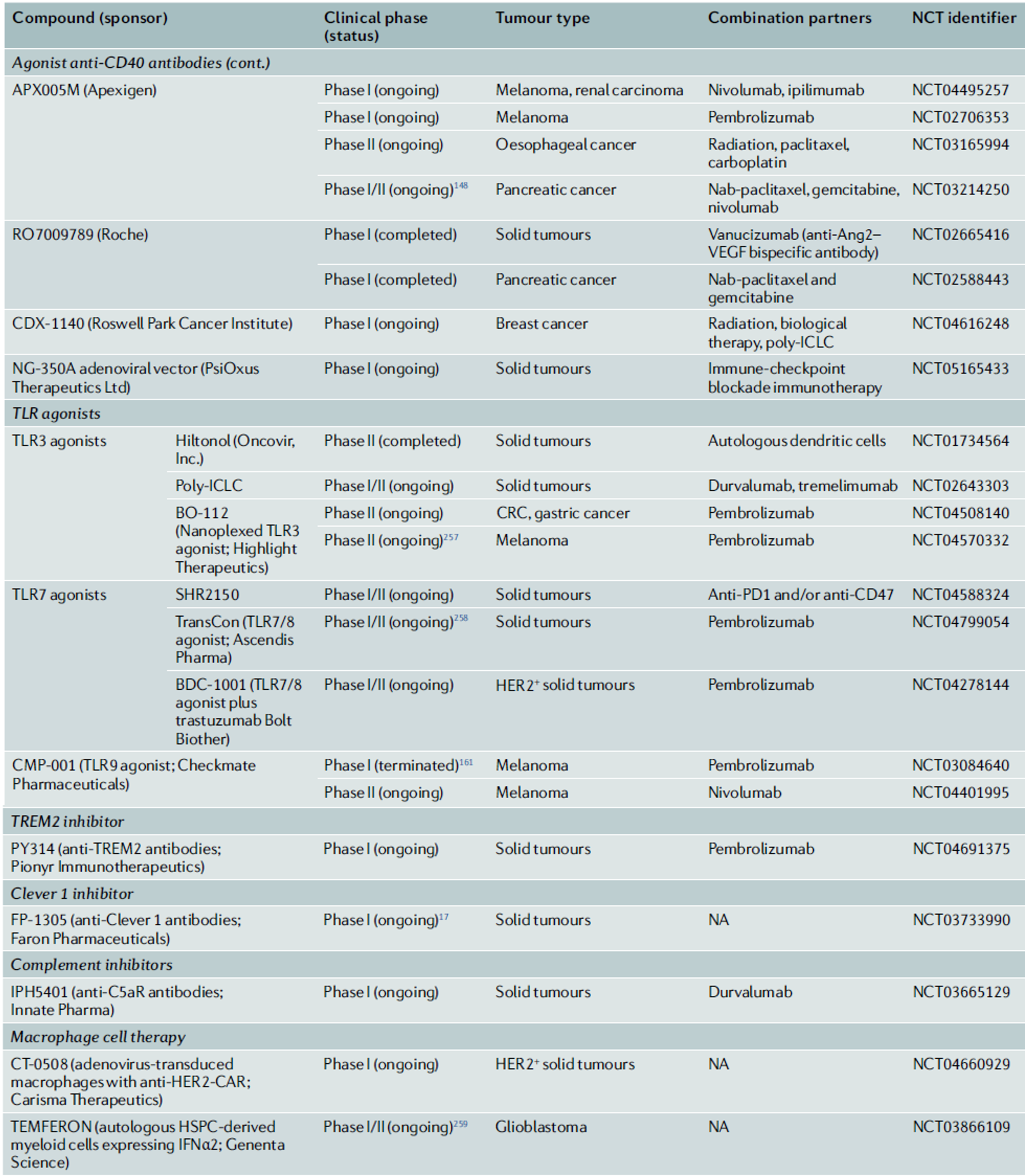
◈ TAMs in Conventional Cancer Therapies
The conventional cancer therapies currently in use include chemotherapy, antibody therapies, and hormone therapies. Upon closer examination of the mechanisms of action of these therapies, macrophages play a key role.
The chemotherapeutic agent doxorubicin induces the secretion of tumor antigens and adjuvants, allowing macrophages to trigger immunogenic cell death (ICD) in tumor cells. Trabectedin, a drug derived from marine organisms, and its analogue lurbinectedin, used as a treatment for non-small cell lung cancer, selectively eliminate monocytes and TAMs, enhancing the effectiveness of cancer therapy.
On the other hand, TAMs can also limit the effectiveness of chemotherapeutic agents. In the case of microglia in brain tumors, TAMs activate the STAT3-MYC pathway within the tumor, leading to resistance to temozolomide. To prevent this resistance, combining anti-CSF1 and anti-CSF1R antibodies, which block CSF1—a factor involved in macrophage differentiation and training—has been shown to have a synergistic effect with chemotherapy in clinical settings.
TAMs also influence the efficacy of antibody therapies. TAMs expressing FcγR can aid in the killing of tumor cells. Antibody therapies such as rituximab (anti-CD20), trastuzumab (anti-HER2), cetuximab (anti-EGFR), and daratumumab (anti-CD38) operate through this mechanism. Even if they do not directly contribute to tumor cell death, antibody therapies can also be used to inhibit angiogenesis. VEGF inhibitors, for instance, can remodel the phenotype of TAMs that secrete VEGF, which drives angiogenesis, thereby inhibiting the formation of new blood vessels and impeding tumor growth.
Hormone-based cancer therapies are also influenced by the inflammatory signaling pathways regulated by TAMs. Inflammatory cytokines like IL-1 and IL-6 activate the signaling pathways via estrogen receptors on cancer cells, leading to tumor growth and inflammation. Androgen receptors expressed directly on TAMs, rather than on hormone receptors within tumors, contribute to the conversion of TAMs into tumor-promoting phenotypes. Thus, anti-androgen therapies can reduce the number of TAMs in tumors, and blocking IL-23 secreted by TAMs can improve the response to androgen blockade therapies.
As demonstrated, even in traditional chemotherapy, anti-angiogenesis therapies, and hormone therapies, TAMs, while not the direct target, significantly impact therapeutic outcomes. The role of TAMs is complex and dual-sided, functioning as both enhancers and inhibitors of tumor development. However, to harness the characteristics of TAMs for actual therapeutic use, a clear understanding of the processes involved in their phenotypic switching and differentiation is necessary.
◈ TAMs in Immune-Checkpoint Inhibitor (ICB) Therapies
With the discovery of the mechanisms of T-cell-mediated immune responses, immune-checkpoint blockade (ICB) therapies have been developed as a basis for immunotherapy. As key regulators of immunosuppressive mechanisms, TAMs play a crucial role in determining the prognosis of ICB and are also extrinsic factors that determine resistance to treatment. This is because they express immune checkpoint molecules such as PDL1, PDL2, CD80 (B7-1), CD86 (B7-2), and PVR. The expression of PD1 by TAMs negatively correlates with their phagocytic activity against tumor cells. Interestingly, TAMs are also known to express receptors that can interact with negative regulators expressed by T cells and NK cells. For example, VISTA, expressed on TAMs, interacts with PSGL1 to function as an immune checkpoint inhibitor on T cells. Therefore, the use of anti-VISTA monoclonal antibodies can lead to changes in TAM transcriptomes and functions, increasing antigen presentation, functional activation, and immune cell migration.
The role of TAMs in ICB therapies has been confirmed in various tissue-specific cancers. For example, neutrophils and TAMs have been found to determine resistance to ICB in triple-negative breast cancer, while TAMs in liver cancer act as negative regulators of T-cell-mediated immunotherapy responses. The pleural and peritoneal cavities are typical examples of environments where tumors progress under immunosuppressive conditions. In these cases, TIM4+ macrophages present in the patient’s body cavity suppress the response of CD8+ T cells, thereby defending against the effects of ICB. Therefore, the removal of TAMs has been shown to reduce resistance to various immune therapy strategies, including vaccines and immune checkpoint inhibitors, and enhance therapeutic efficacy.
In this article, we have explored the role of macrophages present in the tumor microenvironment and their impact on the prognosis of conventional cancer therapies. In the next article, we will delve into therapeutic approaches that directly target macrophages and the macrophage mechanisms that can be leveraged in these approaches.
Reference : Mantovani A, Allavena P, Marchesi F, Garlanda C. Macrophages as tools and targets in cancer therapy. Nat Rev Drug Discov. 2022 Nov;21(11):799-820. doi: 10.1038/s41573-022-00520-5.