Over the years, various cancer treatments have been researched and developed, yet an effective therapy to completely conquer solid tumors has not been established. In the case of solid tumors, they often form a unique environment called the tumor microenvironment (TME), where the surrounding tissue organizes around the tumor. The TME supports tumor growth and metastasis to other tissues through various immune cells and their physical and chemical interactions. As a result, recent cancer treatment approaches have focused on targeting this TME.
In the previous article, we explored the types and roles of tumor-associated macrophages (TAMs), one of the key components of the TME, as well as how they respond to various cancer therapies. Today, based on the functions and roles of TAMs, we will take a closer look at their characteristics that can be applied to cancer treatments and provide some examples.
First, I will outline the targets that can inhibit or reduce TAMs, which possess immunosuppressive characteristics and promote tumor growth within the TME.
- Targeting TAM recruitment and accumulation
Chemokines and CSF1 in tumors are known to recruit TAMs to the tumor site and contribute to determining their function. However, despite successful preclinical data, clinical trials targeting chemokines have been discontinued due to a lack of efficacy in both monotherapy and combination therapies.
On the other hand, approaches targeting CSF1 and its receptor CSF1R have shown high therapeutic efficacy. Numerous preclinical studies have reported that CSF1R inhibitors reduce the number of TAMs in the TME and enhance the responsiveness to chemotherapy. In addition to reducing TAM density, these inhibitors have induced changes in TAM phenotypes and gene expression, as well as increased lymphocyte activation. Specifically, the small-molecule inhibitor BLZ945 has been shown to reorganize the TME through TAMs, NK cells, T cells, and dendritic cells, maximizing the therapeutic efficacy of anticancer agents. However, solid tumors with low CSF1 expression did not exhibit significant effects. Based on these findings, combination therapies using CSF1 inhibitors are currently undergoing clinical evaluation.
Despite these promising results, there are several side effects associated with strategies targeting TAM recruitment. For instance, neutrophils may accumulate compensatorily in the TME as the number of TAMs decreases, or the impact on various cells due to the crosstalk within the chemokine system cannot be ignored.
To mitigate these side effects, a CAR-T cell approach has been proposed to reduce TAMs. FRβ (folate receptor-β) and other M2 markers (CD204, CD206, CD163) were used as indicators of immunosuppressive TAMs, and T cells engineered to recognize these markers specifically eliminated immunosuppressive TAMs. As a result, immune responses were reactivated, and tumor growth was inhibited.
However, despite these efforts, a major limitation remains in targeting the many diverse types of TAMs. Thus, there is an increasing need for precise classification and targeting of TAMs based on a more detailed understanding of their roles.
- Complement
The complement system is a component of the innate immune system and is primarily associated with the mechanisms of inflammation. However, as the relationship between inflammation and tumor growth has become clearer, the complement system has emerged as an important therapeutic target from an oncology perspective. The complement system induces inflammation through three pathways (classical pathway, lectin pathway, and alternative pathway) by hydrolyzing proteins. When the complement system is activated, it leads to the release of anaphylatoxins and the formation of membrane attack complexes.
Anaphylatoxins attract myeloid-derived suppressor cells to the tumor, creating an inflammatory environment conducive to tumor growth. Particularly, when the C3a-C3aR axis of the lectin pathway is activated, it promotes immunosuppressive responses and recruits TAMs. For example, in squamous cell carcinoma, TAMs regulate the secretion of C3, which inhibits cytotoxic T cells and enhances the tumorigenic properties of mast cells and macrophages. Additionally, the alternative pathway has been shown to trigger the infiltration of TAMs into tumors when its regulatory components are deficient, leading to the accumulation of complement activators in the blood.
Complement components can be applied not only as therapeutic targets but also as diagnostic and prognostic markers. Different complement system components have demonstrated potential as prognostic biomarkers depending on the type of cancer, and the degree of complement-related gene expression has also been reported to serve as a predictive indicator of prognosis. Complement components have been found to be associated not only with TAMs but also with T-cell exhaustion, offering a wide range of possible therapeutic applications.
Interestingly, simultaneous inhibition of the complement system and the PD1-PDL1 immune checkpoint axis has shown significant therapeutic effects. This effect is attributed to an increase in CD8+ T cells and a reduction in tumor-infiltrating myeloid-derived suppressor cells, thereby restoring normal immune function. Thus, targeting the complement system could be a promising therapeutic approach, both as a monotherapy and in combination with immune checkpoint inhibitors.
- Inflammasome and IL-1
IL-1 typically triggers immune responses to protect the body, but within the tumor ecosystem, it is associated with tumorigenesis and metastasis. IL-1 promotes the generation and recruitment of immunosuppressive cells, enhances the immunosuppressive functions of TAMs, and consequently suppresses tumor-related immune responses. Based on this understanding, studies have shown that combining immune checkpoint inhibitors with anti-IL-1 antibodies in kidney cancer models yielded enhanced anti-tumor effects. In lung cancer, the use of an anti-IL-1β antibody dramatically reduced cancer incidence and mortality by over 60%. This is likely due to the reactivation of innate and adaptive immune responses when IL-1 signaling is blocked, which enhances anti-tumor effects.
IL-1 can be directly targeted with monoclonal antibodies like canakinumab, but it can also be indirectly targeted by inhibiting its upstream regulator, the inflammasome. Blocking NLRP3, a component of the inflammasome, prevented the reduction of T cells in the tumor microenvironment (TME) and restricted tumor growth. Similar to previous approaches, co-administration with anti-PD1 antibodies resulted in synergistic effects. These findings suggest that modulating inflammatory signaling pathways offers a novel approach to cancer treatment.
- mRNA and microRNA
Currently, mRNA is gaining attention as a key tool for the treatment of various diseases. Formulated through nanodrug delivery systems, mRNA therapies have been used to induce genetic modifications in TAMs, giving them antitumor characteristics. For example, when mRNA encoding IRF5 and IKKβ was delivered via nanoparticles, it was observed that the immunosuppressive TME was altered, and functional changes in TAMs led to tumor cell death.
Unlike mRNA, microRNA (miRNA) does not participate in protein production but plays a crucial role in post-transcriptional gene regulation. It specifically regulates the differentiation of macrophages, including TAMs. When miRNA-155 was delivered to TAMs through mannose-conjugated nanoparticles, it was shown to specifically target TAMs and demonstrate antitumor effects. Interestingly, when the gene for the miRNA-processing enzyme Dicer1 was deleted in macrophages, the IFNγ-STAT1 signaling pathway, which reverses TAMs’ immunosuppressive properties, was activated.
So far, we have explored therapeutic targets that achieve antitumor effects by deactivating or reducing the number of TAMs. However, there are also strategies that aim to activate macrophages to achieve similar outcomes. Specifically, the M1 type of macrophages is known to induce tumor cell death, so we will now look into targets that can stimulate the differentiation of macrophages into the M1 phenotype.
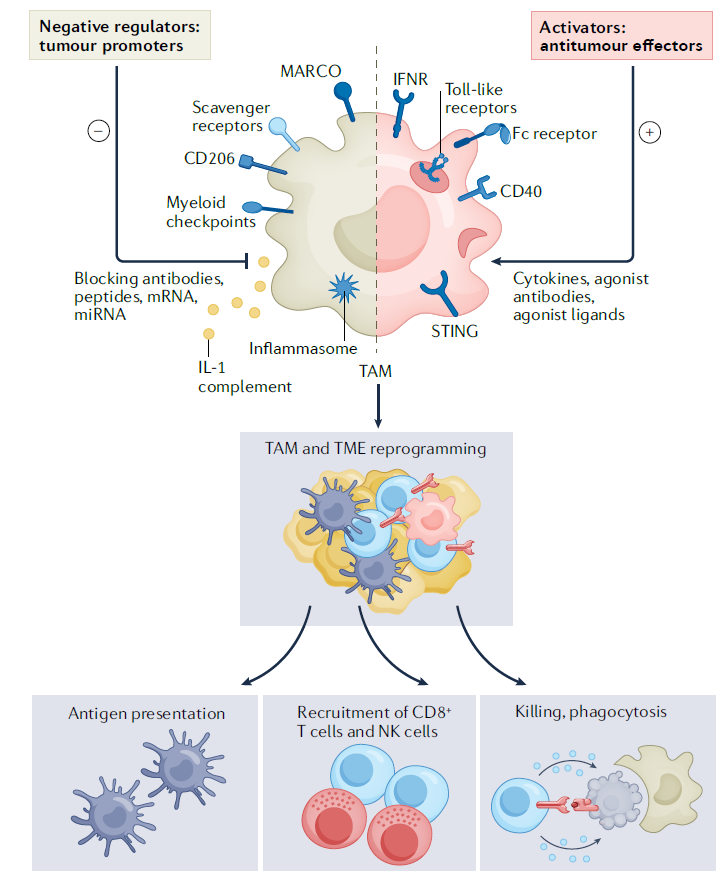
- CD40
CD40 is a TNF receptor expressed on antigen-presenting cells (APCs) and macrophages. When it binds to CD40L on the surface of CD4+ T cells, it becomes activated, leading to the production of TNF, ROS, and reactive nitrogen species. This activation enhances the cytotoxic abilities of TAMs and their ability to suppress tumor growth, while also activating APCs via T cells.
In preclinical studies, agonist antibodies targeting CD40 have shown to reprogram immunosuppressive TAMs, enabling them to gain cytotoxic effects and inhibit tumor growth. Additionally, another mechanism observed with CD40 agonists is the increased infiltration of CD4+ T cells into the TME due to CCL5 secreted by stimulated TAMs. This amplifies the antigen-presenting function of macrophages, further enhancing the immune response against tumors.
- Toll-like receptor
Stimulation of toll-like receptors (TLRs) activates immune responses, which can be leveraged to bypass the immunosuppressive functions of macrophages in tumors and induce tumor cell death. When certain TLR agonists are used, they can reprogram TAMs, enhancing their cytotoxic functions and increasing the secretion of immune-activating cytokines. The first TLR agonist approved by the FDA was BCG (Bacillus Calmette-Guerin), which stimulates TLR2 and TLR4, using an attenuated strain of Mycobacterium bovis. Other successful examples include MPLA(monophosphoryl lipid A).
TLRs 3, 7, 8, and 9, located in endosomes, act primarily as sensors for nucleic acids. When stimulated, these TLRs activate the inflammatory transcription factor NF-kB and promote the secretion of immune-activating cytokines, including type 1 interferons. One notable example is the TLR7 agonist imiquimod, which is the only TLR agonist FDA-approved for local use in non-small cell lung cancer and basal cell carcinoma. Derivatives of imiquimod, such as resiquimod, R848, and motolimod, have also shown promising preclinical results. Another example, the TLR3 agonist Poly I:C shows significant anticancer potential when formulated as a nanocomplex with polyethylenimine (BO-112) and is currently in clinical evaluation.
However, systemic administration of TLR agonists can lead to adverse inflammatory toxicity, so their use is primarily recommended for cancers where direct tumor injection is possible. Various TLR agonists are undergoing clinical trials (phases 1-3), both as monotherapies and in combination with other treatments, and have demonstrated efficacy. Additionally, they are used as adjuvants in cancer vaccines, transforming immunologically “cold” tumors into “hot” tumors, thereby enhancing the effectiveness of immune checkpoint inhibitors when used in combination therapies.
- STING
STING (Stimulator of Interferon Genes) is a key protein that detects damaged DNA or pathogen-derived nucleic acids within cells, triggering the production of type 1 interferons. Given its central role in initiating antitumor immune responses, the development of STING agonists has been actively pursued. A notable STING agonist, DMXAA (5,6-dimethylxanthenone-4-acetic acid), exerts antitumor effects by stimulating STING to promote the secretion of interferons and TNF. Although STING agonists are not macrophage-specific, much of their therapeutic efficacy appears to be linked to the infiltration of macrophages and dendritic cells into tumors.
During early stages of STING agonist development, short half-lives posed challenges for clinical application. However, newer compounds with improved half-lives have since been discovered. While STING agonists were initially administered intratumorally, recent studies on orally-administered STING agonists in mice have shown promising results, particularly when used in combination therapies. Moreover, STING has also been implicated in responses to microbial metabolites like c-di-AMP. Research suggests that a high-fiber diet, which modulates the gut microbiota, could enhance the efficacy of immune checkpoint inhibitors via STING-related pathways.
In this text, we have explored various targets aimed at deactivating or reducing immunosuppressive TAMs or promoting their differentiation into M1 macrophages, which exhibit antitumor properties. Macrophages are unique in their plasticity, capable of differentiating into various subtypes depending on the stimuli they encounter. Thus, a deep understanding of these complex mechanisms is essential for their effective therapeutic use. However, given the critical role that macrophages play in the tumor microenvironment (TME), efficiently modulating their activity holds significant potential for developing successful anticancer strategies.
Reference : Mantovani A, Allavena P, Marchesi F, Garlanda C. Macrophages as tools and targets in cancer therapy. Nat Rev Drug Discov. 2022 Nov;21(11):799-820. doi: 10.1038/s41573-022-00520-5.