Despite the development of numerous new drugs and therapeutic approaches for various neurological disorders, effective treatments remain elusive, primarily due to the presence of the blood-brain barrier (BBB). Unlike typical vascular structures, the BBB is a highly selective and sophisticated barrier designed to protect the brain by strictly regulating the passage of substances. Consequently, most drugs cannot cross the BBB, making the development of effective drug delivery strategies that can bypass or traverse this barrier essential—particularly those utilizing non-invasive methods, which are currently the focus of intensive research.
One promising approach gaining attention is the use of extracellular vesicles (EVs) as drug delivery vehicles. EVs not only appear capable of traversing the BBB under relatively natural conditions, but they may also influence the structural integrity of the BBB itself. However, the molecular mechanisms underlying these properties remain incompletely understood. This article aims to summarize the key pathways by which EVs are thought to cross the BBB, based on current research, and to explore strategies for their application in the treatment of brain disorders.
1. Structural characteristics of the BBB
The BBB is a complex structure formed by the coordinated interactions of multiple cell types. Its core component is the brain endothelial cell, which forms the vascular lining and is interconnected via tight junctions, adherens junctions, and gap junctions, establishing a robust barrier. Surrounding these endothelial cells are pericytes, which support vascular stability, and the basement membrane, which provides structural integrity. Astrocytic endfeet enwrap the outer surface of the vessels, completing the BBB’s architecture. This multilayered structure serves to restrict and selectively regulate the passage of molecules into the brain.
2. Mechanisms of EV translocation across the BBB
To cross the BBB, substances must utilize either passive diffusion or active transport. Passive diffusion is typically limited to small molecules under 500 Da with high lipid solubility. Larger biomolecules and most drugs must rely on active transport mechanisms, which include carrier-mediated transport, efflux pumps, receptor-mediated transport, and adsorptive-mediated transcytosis.
The ability of EVs to cross the BBB has been demonstrated in multiple studies, with transcytosis being the most plausible route. Five key mechanisms have been proposed for EV transcytosis across the BBB:
- GPCR-mediated internalization: EVs bind to G-protein-coupled receptors, initiating signaling cascades that facilitate vesicle uptake.
- Endocytosis of EV cargo: Internalization of EV components triggers intracellular signaling within endothelial cells.
- Micropinocytosis: EVs induce invagination of the cell membrane to form new vesicles.
- Receptor-mediated transcytosis: EVs are directed into multivesicular bodies (MVBs) for intracellular transport.
- Lipid raft-dependent endocytosis: EV uptake is modulated via lipid raft microdomains.
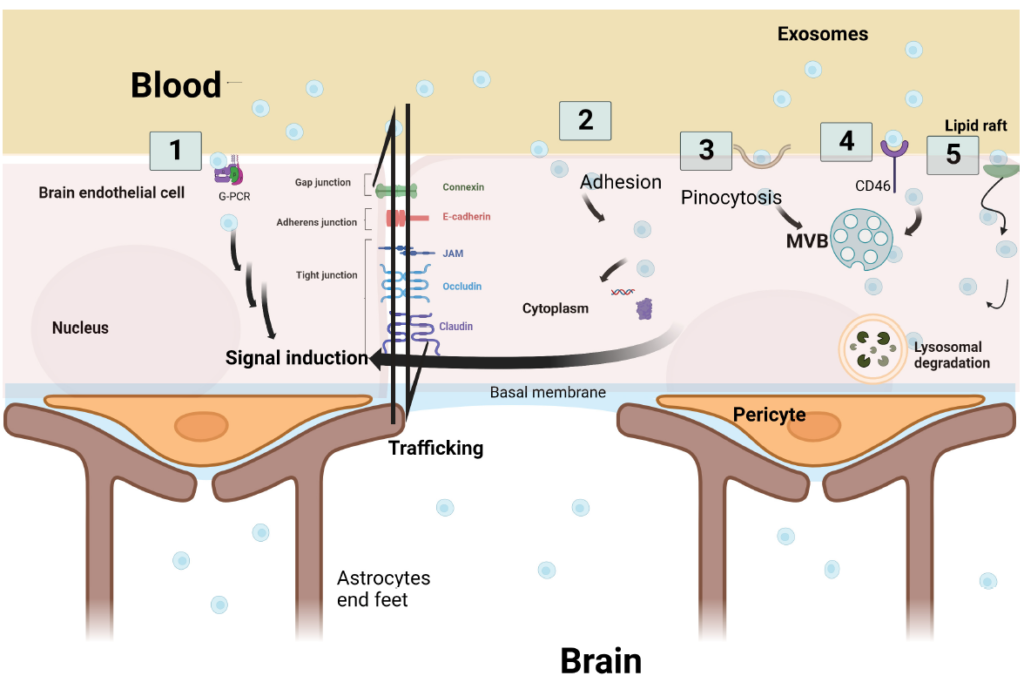
Once inside the endothelial cell, EVs may undergo one of three fates: lysosomal degradation, cytosolic release of signaling molecules, or re-packaging into intraluminal vesicles (ILVs) and exocytosis.
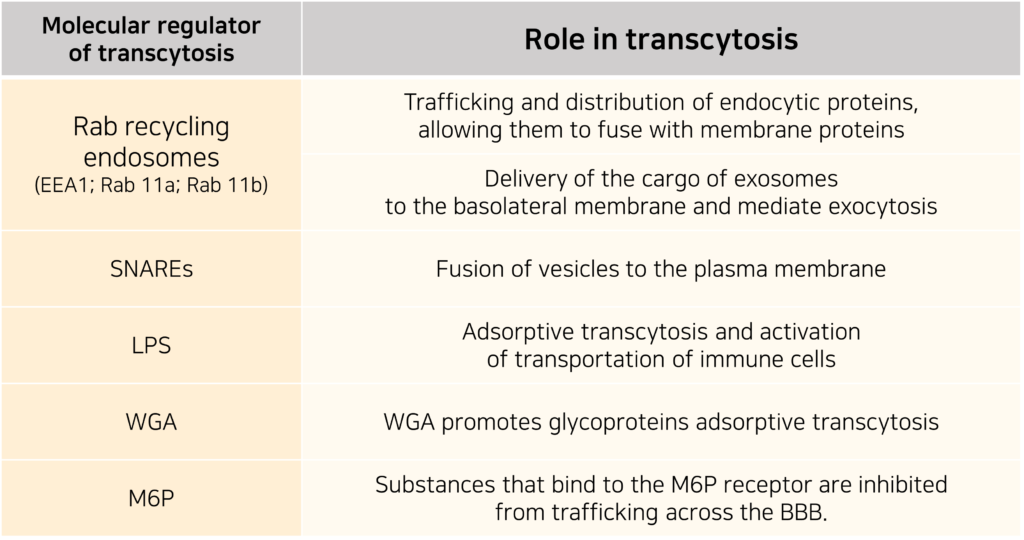
Fluorescence-based analyses have shown that EVs interact with the endothelial membrane to deliver their cargo into the cytoplasm, and that this process varies depending on the EVs’ size and density.
Inflammatory conditions significantly enhance EV translocation across the BBB. Inflammation disrupts the BBB’s structural stability and promotes EV endocytosis. Hypoxic conditions, commonly seen in inflamed tissue, increase intracellular calcium levels in endothelial cells, weakening junctional proteins and facilitating EV passage. This mechanism may explain both the influx of circulating EVs into the brain and the efflux of brain-derived EVs into the peripheral circulation, supporting their potential as biomarkers for neurological diseases.
Proinflammatory cytokines such as IL-6 and TNF-α have been shown to increase BBB permeability to EVs. Furthermore, in pathological contexts such as tumors or stroke, EV translocation is activated via receptor-mediated or lipid raft-dependent endocytic pathways.
3. Therapeutic Application of EVs in Neurological Diseases
EVs are emerging as promising drug carriers. Some EVs possess inherent therapeutic properties—such as those derived from stem cells—while others are bioengineered for improved production, purification, drug loading, stability, and quality control. Compared to other nanocarriers, EVs offer advantages including targeted delivery, biocompatibility, safety, tissue adhesion, bioavailability, and uptake efficiency, making them an attractive subject of ongoing research.
The ability of EVs to cross the BBB greatly enhances their therapeutic potential for central nervous system (CNS) diseases. For example, stem cell-derived EVs are being developed as novel treatments for traumatic brain injury. These EVs contain microRNAs (miRNAs) capable of regulating gene expression in target cells post-transcriptionally, promoting tissue regeneration. They also exhibit long-term stability in storage. By encapsulating anti-inflammatory agents or growth factors, engineered EVs could potentially be used to treat anxiety disorders, treatment-resistant depression, and neurodegenerative diseases.
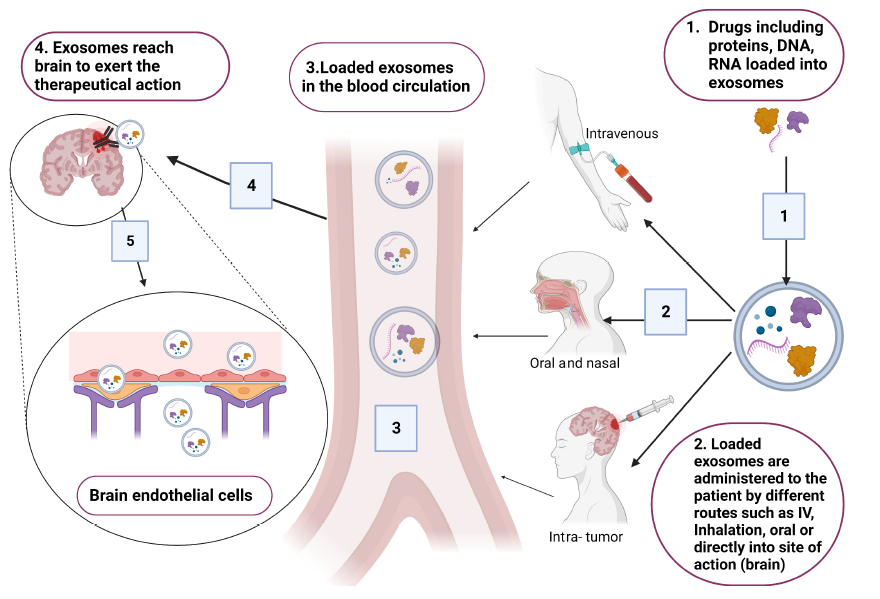
Stem cell-derived EVs, particularly those from mesenchymal stem cells (MSCs) or induced pluripotent stem cells (iPSCs), are especially promising in regenerative medicine due to their lack of immunogenicity and their non-invasive delivery routes, contrasting with traditional invasive therapies. Various applications of stem cell-derived EVs in neurological disease models have been documented.
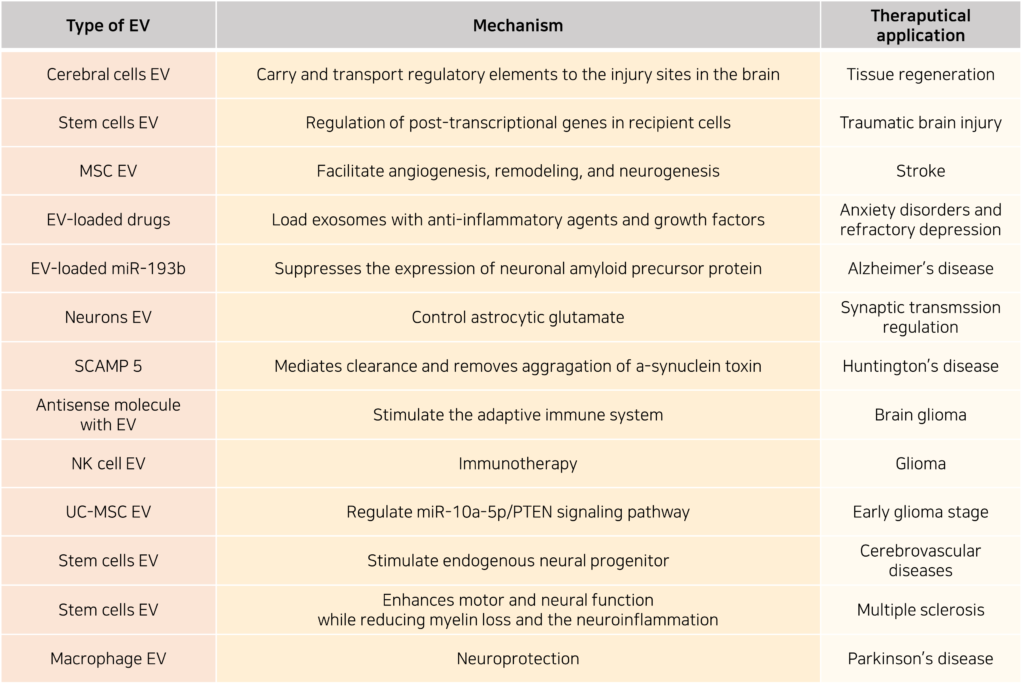
Despite these advantages, several challenges remain before EV-based therapies can be translated into clinical practice. These include high production costs, low purification yields, and potential aggregation during manufacturing, which can compromise stability and uptake efficiency. Furthermore, the heterogeneity of EV cargo poses a risk of eliciting immune responses.
Nevertheless, given their numerous strengths, EVs hold considerable promise as a revolutionary therapeutic platform for CNS diseases—provided that technical hurdles related to large-scale production and purification are adequately addressed.
Reference : Abdelsalam M, Ahmed M, Osaid Z, Hamoudi R, Harati R. Insights into Exosome Transport through the Blood-Brain Barrier and the Potential Therapeutical Applications in Brain Diseases. Pharmaceuticals (Basel). 2023 Apr 10;16(4):571. doi: 10.3390/ph16040571.