In the previous three articles, we discussed various types of immune oncology therapies and their mechanisms of action. Concluding the introduction to immune oncology therapies with the topic of cancer vaccines, we will explore the research and development directions of immune oncology therapies.
Cancer vaccines
Cancer vaccines induce immune system responses to protect our bodies from cancer. Cancer vaccines can be categorized into preventive and therapeutic approaches. Preventive cancer vaccines employ a concept similar to traditional vaccines, primarily administered to prevent cancers caused by viruses. Notably, preventive cancer vaccines against Hepatitis B and human papillomavirus have played a crucial role in reducing the incidence of liver cancer and cervical cancer. On the other hand, therapeutic cancer vaccines are developed with the aim of amplifying immune responses to eliminate cells that have already transformed into cancerous cells. Early models of therapeutic cancer vaccines included vaccines containing Mycobacterium bovis, originally used for tuberculosis prevention but later repurposed as initial treatment vaccines for bladder cancer.
The discovery of tumor-associated antigens (TAAs) paved the way for therapeutic cancer vaccine approaches. However, TAAs often fail to induce appropriate immune responses as they are recognized as “self” by the immune system. Therefore, virus antigens or neoantigens specifically expressed in malignant tumors became suitable targets for cancer vaccines.
Vaccines in the 1970s were developed based on autologous tumor vaccines. These vaccines, administered along with adjuvants or viruses, aimed to elicit complex responses against TAAs obtained from patient-derived cancer cells. Additionally, synergistic effects observed when combining autologous tissue tumor vaccines with immune checkpoint inhibitors were observed in malignant melanoma. Various autologous tumor vaccines are currently undergoing phase 2 and 3 clinical trials. However, this approach to cancer vaccines faces limitations in obtaining patient-derived cancer cells for specific cancer types. To overcome this challenge, personalized recombinant cancer vaccines based on advances in NGS technology for tumor-derived DNA have emerged.
Development of personalized recombinant cancer vaccines
Vaccines that induce responses to tumor-derived neoantigens are expected to elicit more potent immune responses compared to cancer vaccines based on tumor-associated antigens (TAAs). Furthermore, T cells activated by such vaccines, targeting neoantigens, are predicted to have no toxicity due to autoimmune reactions as they target T cells that have not undergone negative selection processes. Additionally, with advancements in research on tumor-derived DNA, there has been increased understanding, demonstrating the clinical potential of personalized cancer vaccines targeting neoantigens.
However, due to the high rate of mutations in tumors and the fact that only a small proportion of neoantigens can induce meaningful responses in patients, considerable time and effort are required to identify appropriate neoantigens. Attempts to increase the efficiency of this process have been made, particularly using next-generation sequencing (NGS) technology to discover significant neoantigens. In the study, out of 50 neoantigens, 16 significant ones were identified, with many of them inducing cytokine responses more effectively in CD4+ T cells than CD8+ T cells, suggesting that neoantigens are selected through MHC class II.
To design and produce personalized vaccines for actual therapy, computer-based algorithms are used to determine which tumor-derived peptides will form suitable TAAs or tumor neoantigens for the patient’s MHC. Once the responsiveness of neoantigens is confirmed, various strategies can be chosen to manufacture vaccines based on these antigens, including synthetic peptides, mRNA, virus and DNA plasmids, and antigen-loaded dendritic cells. However, the direct impact of each method on immunogenicity has not been directly compared.
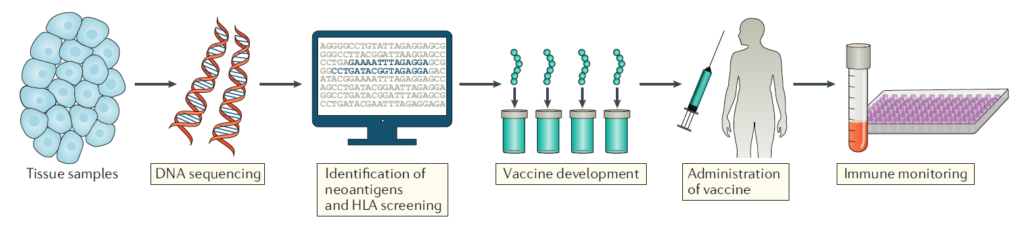
Pitfalls and adverse effects of cancer vaccines
Cancer vaccine research is a promising field, but it still faces significant challenges. Each individual tumor can have thousands of somatic cell mutations, making it extremely difficult to predict which neoantigens will produce potent anti-cancer effects. However, methods such as measuring the expression of mutated mRNA or predicting peptide-MHC binding through software databases have shown remarkable effects in clinical settings. These successful clinical cases have targeted MHC class 1-specific neoantigens, while targeting MHC class 2 still poses difficulties.
Simultaneously, a concern is the time and cost involved in developing personalized vaccines. Currently, it takes about 4 months to develop such vaccines, highlighting the importance of shortening the development time, particularly for rapidly progressing metastatic cancers.
Furthermore, clinical trials for cancer vaccines are still in early stages, emphasizing the need to focus on the results of future clinical trials to draw definitive conclusions.
Nevertheless, cancer vaccines represent a noteworthy approach as they do not treat patients with the same cancer type identically but recognize each patient’s disease as an independent entity, paving the way for a “single patient and single disease” treatment approach. Future research and technological advancements are expected to address the current limitations facing cancer vaccines.
Emerging cancer immunotherapeutics
The high frequency of genetic alterations in cancer and their infinite variety add complexity to the mechanisms of cancer, making treatment challenging. However, rapid advancements in the field of immune oncology have led to the proposal of therapeutic approaches that can surpass the complexity of cancer through the addition of various modifications to current immunotherapies.
As the first alternative, combination therapy is being explored. Successful cases in clinical trials have shown that combining therapies acting through different mechanisms can be successful in treating various types of cancer. For example, the combination therapy of ipilimumab and nivolumab has shown efficacy in metastatic melanoma and renal cell carcinoma, receiving FDA approval. The synergy between anti-CTLA4 and anti-PD1 therapies stems from the complementary action of CTLA4 and PD1. The cross-talk of the CTLA4 and PD1 signaling pathways mediated by CD80 and PDL1 heterodimerization serves as a basis for explaining the mechanism by which combination therapy successfully treats cancer. However, the combination of immune checkpoint inhibitors may increase the risk of drug toxicity.
In addition to dual therapies, combining radiation therapy with immune checkpoint inhibitors is another new approach for refractory cancers. The immunomodulatory effects of radiation therapy alone are two-sided. Mechanistically, radiation therapy suppresses immune responses by inducing PD1 expression on tumor cells while increasing the diversity of anti-tumor responses by exposing neoantigens. Therefore, based on preclinical results, the combination effect of radiation therapy and PD1 inhibitors is expected to be a highly attractive treatment, particularly for patients with metastatic cancer.
Unlike combination therapies that combine existing treatment methods, research is also underway to identify new targets for anticancer therapy. Targets such as LAG3 (Lymphocyte activation gene 3), TIM3 (T cell immunoglobulin 3), VISTA (V-domain immunoglobulin suppressor of T cell activation), B7-H3, and TIGIT (T cell immunoreceptor with immunoglobulin and immunoreceptor tyrosine-based inhibitory motif domains) are being investigated as new targets for T cell activation. For example, LAG3 acts to inhibit T cell activation and is used as a marker for T cell exhaustion. Inhibition of LAG3 signaling using LAG3-Ig fusion proteins or monoclonal antibodies has shown efficacy when combined with paclitaxel in breast cancer, demonstrating therapeutic efficacy in 50% of patients. In addition to immune checkpoint mechanisms, targets such as ICOS (Inducible co-stimulator) on T cells are being explored to enhance the efficacy of existing immune oncology therapies, with preclinical or early clinical stages of development underway.
Conclusion
Efforts to treat cancer by targeting T cells have steadily evolved. While it took a long time for the transition from basic science to clinical medicine to apply this treatment method, eventually, it led to the modulation of the immune system to treat cancer. Future research is expected to focus not only on T cells but also on regulating antigen-presenting cells (APCs) and NK cells as approaches to treatment.
In refractory tumors, T cell-based therapies and cancer vaccines have surpassed the treatment scope of traditional chemotherapy, which has been the most effective treatment so far. Although side effects related to immune reactions still exist, innovative therapies utilizing immune responses are expected to be applied more smoothly than conventional chemotherapy. Fueled by successful treatment cases, immune oncology therapies will continue to be researched and developed, finding new targets and advancing further.
Reference : Waldman AD, Fritz JM, Lenardo MJ. A guide to cancer immunotherapy: from T cell basic science to clinical practice. Nat Rev Immunol. 2020 Nov;20(11):651-668. doi: 10.1038/s41577-020-0306-5.