The facilities, management practices, and testing processes in biopharmaceutical production have evolved to prevent the risk of virus contamination in the drug manufacturing process. An example of virus contamination in pharmaceuticals is the case where hepatitis viruses or HIV viruses were transmitted to humans through plasma products in the 1980s and 1990s. During that time, there were no virus removal steps in the plasma product processing, and there was no virus screening method for blood donors, resulting in many deaths.
Biopharmaceutical processes should be capable of monitoring and preventing virus or microbial contamination in real time. However, contamination testing at Good Manufacturing Practice (GMP) levels often takes longer than the process throughput time of the drug, making it difficult to respond immediately to contamination. Additionally, there is a greater risk of contamination substances entering downstream processes that do not significantly affect cell culture stages. Once a virus enters a downstream process of the bioreactor, the contamination scope expands to the entire process, amplifying the scale of disposal. Thoroughly designed downstream processes remove contamination substances across multiple stages, but strict criteria must be applied to ensure the definite removal of contaminants.
In bioreactors, virus testing can be performed rapidly, allowing for swift action before contaminated products move to downstream processes. Analytical methods used at this stage include High-Throughput Sequencing (HTS) and quantitative polymerase chain reaction (qPCR) experiments. While qPCR analysis requires genetic information about the virus being tested, HTS has the advantage of detecting unknown viruses for which genetic information is not available.
Biopharmaceutical manufacturers must design process protocols to prepare for and prevent infections by microorganisms such as viruses, fungi, and bacteria. The items listed in the table below can serve as examples of such protocols.
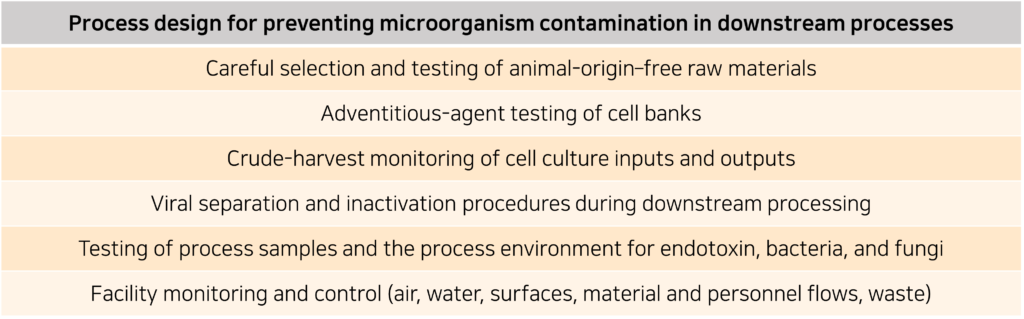
Assessing viral clearance in downstream processes
Evaluation of viral clearance (VC) in downstream processes is an important safety requirement for biopharmaceuticals. ICH 5A guidelines mandate the evaluation of VC for model viruses or viruses of concern for infectivity. For example, in the plasma product industry, testing for hepatitis C virus (HCV) is conducted for human-derived materials.
VC evaluation assesses the removal of retrovirus-like particles (RVLPs) that may occur during downstream processes of mammalian cell cultures. This assessment yields an RVLP safety factor, comparing the theoretical amount of retrovirus in product doses, evaluating whether virus removal is appropriately accomplished. VC evaluation also serves as an indicator of process robustness by assessing how effectively the process removes various types of viruses with different physicochemical properties. Typically, VC evaluation is outsourced to specialized firms, although some companies may conduct it internally.
The guidelines of ICH, PDA, BPOG, and ISPE all mention the necessity of VC evaluation and provide methodologies and approaches for interpreting the evaluation results. Typically, minute virus of mice (MVM) and xenotropic murine leukemia virus-related virus (X-MuLV) are used as models for non-enveloped and enveloped viruses, respectively, in early-stage clinical VC evaluations. For later-stage clinical VC evaluations, a minimum of four virus models are required, typically including X-MuLV and MVM along with 2-3 additional virus models. The selection of virus models for VC evaluation on chromatography resins is based on factors such as the presence of envelopes, and whether the virus is DNA or RNA-based. Other viruses that can be used include reovirus type 3, bovine viral diarrhea virus (BVDV), vesicular stomatitis virus (VSV), Sindbis virus, pseudorabies virus (PRV), among others.
Simplifying VC studies using virus substitutes
Regardless of the amount of virus present in a drug, pharmaceutical companies must discard contaminated raw materials. What VC evaluation provides is assurance that even if the process is exposed to viruses during operation, they will not be transmitted to patients. Current biopharmaceutical production processes include steps for inactivating enveloped viruses, which may involve strong acid reagents, detergents, high concentrations of arginine, anion exchange chromatography, virus filters, among others.
Generally, contamination tests for viruses, mycoplasma, bacteria, fungi, etc., are conducted on cell culture media before purification, and these results may be confirmed after the production process is completed. In contrast, VC evaluation quickly confirms whether a wide range of virus species are inactivated and removed throughout the entire downstream process.
VC evaluation studies can take several months to complete. Important VC studies can incur costs of millions of dollars and require a significant amount of manpower. However, investing time and resources into VC studies is inevitable to ensure patient safety.
Critical VC studies should be conducted in specialized laboratories with high biosafety levels, testing at least four mammalian viruses. Virus panels in both early and late-stage clinical studies commonly include X-MuLV and MVM, both of which are viruses capable of infecting CHO cells.
However, these viruses are not the only ones used; the following non-mammalian viruses are also used as substitutes for enveloped or non-enveloped viruses:
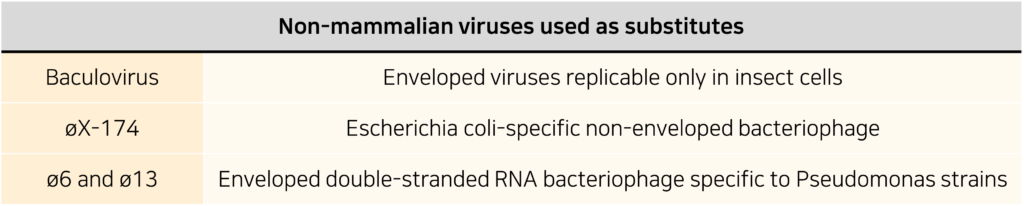
One alternative is to use virus-like particles (VLPs). VLPs are capable of self-assembly and offer the advantage of incorporating one or more virus structural proteins. Depending on the type, VLPs can opt to possess membranes. While they mimic viruses, they do not contain genetic material necessary for host cell infection, making them useful for VC studies.
An alternative virus to MVM, øX-174
MVM and øX-174 are non-enveloped viruses with similar sizes, isoelectric points, and levels of physiochemical resistance (high). øX-174 does not infect mammalian cells and has pharmaceutical resistance similar to enteroviruses and parvoviruses, with higher physiological resistance compared to adenovirus serotype 5 or poliovirus, making it suitable for testing the integrity of biopharmaceuticals.
Considering these characteristics, øX-174 can be used as an alternative to MVM under certain conditions. If the designed process does not allow both viruses to coexist under the same conditions, virus-like particles (VLPs) such as MVM Mock virus particles (MVM-MVP) could be suitable alternatives.
The similar sizes of MVM and øX-174 also suggest their potential use as substitutes in nanofiltration processes. Typically, PP7 and øX-174 are used as substitutes for small mammalian viruses in nanofiltration processes, as they are easier to handle or study than MVM, making them attractive alternatives.
Instead of using four or more complex virus models, VC studies in nanofiltration processes can be simplified by using MVM or MVM analogs. Nanofiltration processes remove viruses based on size, filtering out virus particles larger than the pore size of the membrane. Parvoviruses or small bacteriophages are not suitable models for nanofiltration process evaluation because their sizes are very similar to the standard filter pore size, leading to inaccurate results. Therefore, it is desirable to use the removal rates of MVM, MVM-MVP analogs, øX-174, or other bacteriophages of similar size as the results of VC evaluations.
MVM-MVP, øX-174, and others can also be used as substitutes for MVM in VC chromatography steps. Experiments utilizing them have been introduced into the design of experiments (DoE) to study optimal or worst-case chromatography conditions for VC. Significant results have been obtained in anion exchange chromatography (AEX), hydrophobic interaction chromatography(HIC), Protein A affinity chromatography, and other methods.
Alternatives to X-MuLV : Baculovirus / ø6 and ø13 bacteriophages
Baculovirus (200-450nm) and X-MuLV (80-120nm) exhibit lower drug resistance compared to non-enveloped viruses, but Baculovirus shows higher resistance than X-MuLV to acidic conditions, detergents, and solvent/detergent treatment. It can survive for about 1 hour at pH 3.0 and around 10 minutes at pH 12.0, as well as withstand 90 minutes at 55°C. Baculovirus’s excellent drug resistance makes it a suitable alternative for use in VC studies, and its size is similar to that of PRV (150-200nm), which is commonly used in virus research.
The lytic enveloped bacteriophages ø6 and ø13 have shapes (icosahedral) and sizes (~80-85nm) similar to X-MuLV. Therefore, these phages can be considered as alternatives to X-MuLV in nanofiltration processes. The virion of ø6 is vulnerable to detergents and chloroform and remains stable between pH 6.0 and 9.5. However, there is limited data on the reactivity to detergents, solvents, and pH conditions when they are used in virus inactivation processes required for protein biology.
Physiochemically, ø6 and ø13 have been confirmed as suitable alternatives to X-MuLV in chromatography or virus inactivation steps. Still, it’s essential to consider the possibility that non-infectious RVLPs may be a better alternative.
Controlling adventitious agents in downstream processing
Over the past 20 years, process facilities has advanced, but contamination in processes still occurs. While virus contamination is rare, the cost incurred when contamination does happen can exceed hundreds of billions of dollars, so caution is warranted. Current downstream processes are not fully closed, making it impossible to maintain aseptic conditions throughout the process. However, the overall sterilization process can degrade the properties and performance of chromatography resins, and columns are not completely sealed, so sterilized resins cannot be guaranteed to remain sterile over prolonged use. Therefore, since it is not possible to sterilize or maintain the entire downstream process in a sealed state, the process itself must be conducted in a closed environment or the surrounding environment must be controlled.
The conditions for inactivating numerous non-enveloped viruses are extreme physicochemical conditions that can render proteins unstable. These viruses exhibit resistance to extreme pH, high temperatures, and chemical agents. For example, Parvovirus can survive temperatures of 80-90℃ for up to 10 minutes and can withstand 2500ppm of NaOCl. While NaOCl treatment may not completely inactivate viruses like MVM and poliovirus, it can effectively act on adenovirus and vaccinia. Ethanol, on the other hand, is effective against vaccinia and adenovirus but shows no activity against poliovirus or parvovirus. Therefore, through optimization of process design, it may not be possible to completely inactivate all non-enveloped viruses, and the most efficient approach may involve controlling virus ingress through facility environment management.
Bacterial or fungal contamination occurs more frequently than viral contamination. From 2019 to 2021, pharmaceutical manufacturing facilities recalled 175 batches due to such microorganism contamination. Unlike viruses that cannot proliferate without a host, bacteria and fungi begin to proliferate upon entering the process and are difficult to eradicate. For example, Bacillus strains form heat-resistant spores in organic solvents, allowing them to proliferate even in nutrient-poor environments. These spores typically require heating at 110-130 degrees Celsius for 20-40 minutes for sterilization, but such conditions can destroy proteinaceous products.
Fortunately, many active bacteria and fungi can be eradicated under stable product conditions. Treatment with polyols (such as glycerol, ethylene glycol, and propylene glycol) diluted to 18% or more in buffer solutions can sterilize bacteria and fungi. The antimicrobial effects of polyol solutions are also reported in the United States Pharmacopeia Chapter 51. Additionally, other methods such as preservatives and pH adjustment can be chosen to apply enhanced antimicrobial effects.
Polyols exhibit properties that stabilize proteins and prevent their aggregation or denaturation. However, they can also affect the binding affinity between proteins and ion exchange chromatography resins. Therefore, the introduction of polyols should be comprehensively considered in the design of downstream processes.
Through the text, the importance of analytical methods for virus removal in biopharmaceutical manufacturing processes and the need for effective removal processes have been outlined. Ensuring patient safety and preventing unnecessary time and cost consumption for pharmaceutical companies, efficient and thorough process design is essential.
Reference : Microorganism Contamination in Downstream Biopharmaceutical Processes: A Holistic Approach to Measurement, Control, and Prevention, BioProcess International (https://www.bioprocessintl.com/separation-purification/microorganism-contamination-in-downstream-biopharmaceutical-processes-a-holistic-approach-to-measurement-control-and-prevention)